By Thomas P. Turner
To recover from a stall, your instinct is to use ailerons to raise a dropped wing. But this could result in the aircraft going into a snap roll. What routine should you practice to avoid this result?
I’m in the right seat conducting flight instruction. As the pilot receiving instruction (PRI) begins a practice stall, I move my hands and feet toward the controls. As the stall occurs, I’m ready to intercede if needed in all three axes: elevator, rudder and aileron.
All pilots should know why I’m ready to push forward on the elevator control. Most understand, or at least can parrot back, why I’m concerned about the rudder. But in my experience few pilots think about the vital role aileron control plays in stall recovery and spin avoidance, and how incorrect aileron input can be as bad as stomping on the wrong rudder pedal.
To understand why this is so, and ensure you control all three axes properly at slow speeds and high angles of attack, let’s go back to basics, then build on this level of understanding and action.
Angle of attack
You know the story: angle of attack (AOA) is defined as the angular difference between the chord line of the wing and the relative wind. As you increase AOA, airflow begins to detach from the upper surface of the wing and this turbulent flow reduces lift while it creates drag. Increase AOA beyond some angle—the critical angle of attack—and the wing stalls. A stall can occur at any airspeed and pitch attitude. Got it? But do you really understand it?
Most manuals include a diagram similar to Figure 1. A relatively low AOA, say 10 degrees, results in smooth airflow across the entire top (and bottom) of the wing, generating lift. Pull back on the elevator to increase AOA toward 16 degrees or so and air cannot make the tight turn across the top of the wing; at some point the airflow detaches, reducing lift-generating area and increasing drag. Some additional lift is created by impact with the bottom of the wing and the rebounding force of that impact, so total lift continues to increase. However, at around 17 degrees AOA in most airplanes, airflow detachment is so great that total lift is insufficient to sustain flight. The wing stalls.

The problem with this diagram and the way we usually practice stalls is that they strongly suggest the wing, and therefore the nose of the airplane, must be pointed very high for a stall to occur. And yet we learn the wing can stall at any attitude. How is that so?
A simpler definition may make more sense: angle of attack is the difference between the direction the aircraft is pointed and the direction it is going. If the aircraft is level, climbing or even descending and you pull back on the elevator, it does not instantaneously change direction. Inertia causes it to continue in the direction it was going and then arc into a new direction that, if power or speed is sufficient, will change to approximately the new attitude. To illustrate this, draw an aircraft around the wings in the standard diagram, taking into account the small angle of incidence—slight ‘leading edge up’ of the wing as mounted onto the fuselage. Then rotate the entire diagram to show how the critical AOA can be reached during approach, take-off and go-around (balked landing), while the aircraft’s attitude appears close to normal (Figure 2).
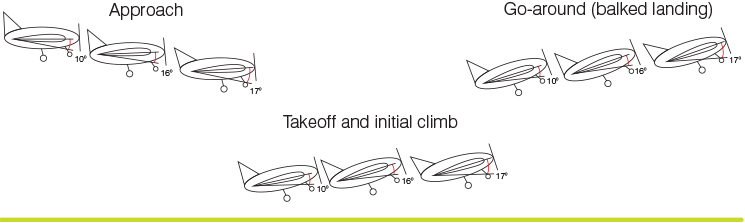
If you make elevator input smoothly and gradually, there is little change in AOA during the transition. Pull aggressively, however, and the AOA change is initially greater because the airplane’s nose changes direction faster than the aircraft can change direction of flight. You ‘load’ the wing. This is why stall speed increases with an increase in G load and may enter an ‘accelerated’ stall. This can happen in a turn (where we usually practice accelerated stalls), but may also occur in wings-level flight in climb and even descent: for instance, if you pull and change the direction the nose is pointed, faster than the aircraft can change its direction of flight.
Bank angle alone does not increase stall speed. If the aircraft’s nose is permitted to pitch downward and G load doesn’t increase, stall speed and AOA do not increase, regardless of bank. Knowing that pulling on the controls against the airplane’s direction of flight increases G load and consequently AOA, makes it easy to understand why pushing forward on the stick or yoke—unloading the wing—is the first, right thing to do in stall recovery.
Angle and lift
Another diagram that has deeper meaning than many pilots are taught is Lift vs. AOA changes (Figure 3). Most instructors focus on the critical AOA at the 17 to 18 degrees point. The stall indeed occurs at the critical angle of attack. But reduce AOA just one degree below critical and the wing is generating its maximum lift. This is a vital concept to understanding the need for aileron control near and at stall AOA.
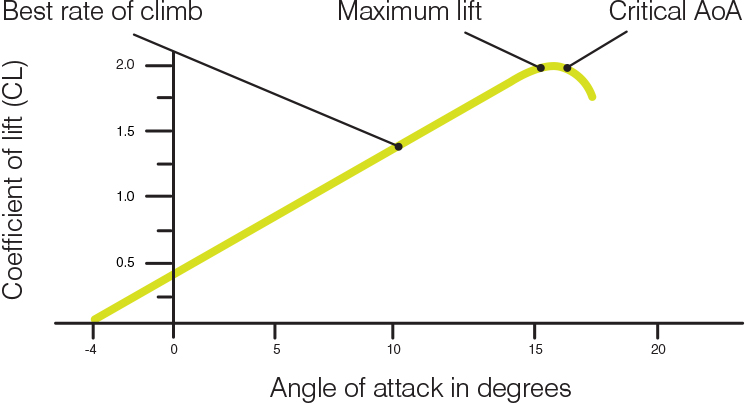
I’ve added the maximum lift AOA and an approximation of AOA for VY speed (best rate of climb) to Figure 3. Also interesting: lift is generated beyond critical AOA—it’s just the lift is less and the drag is so great that the wing can no longer support the airplane. It takes a significant push on the elevator control to get the wing back into flying range and recover from this stall.
Asymmetry
Everything in this article so far assumes the angle of attack is the same on both wings and the aircraft is in coordinated flight. The savvy among you know the reason uncoordinated flight is hazardous at slow speeds and/or increased G load is that when the aircraft is slipping or skidding, angle of attack is not the same on both wings. One wing may stall before the other. Let’s look at how this happens.
Figure 4 shows the balance of force in coordinated (left), slipping (center) and skidding (right) flight. In coordinated flight and assuming one G, the aircraft travels essentially in the direction its nose is pointed. AOA remains constant and is the same on both wings.
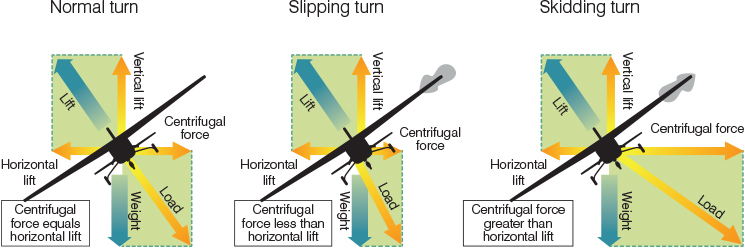
In a slip the tail of the aircraft is displaced—or yawed—toward the outside of a turn, or to one side of a straight flight path if balanced by control input. The outside wing is traveling faster through the air than the inside wing but is also forced into a higher angle of attack. If you decrease speed or increase back pressure (or both), the high wing will stall first. This tends to level the wings and also reduce the outside wing’s angle of attack. A stall out of a slip is only somewhat more hazardous than a coordinated stall.
By contrast, in a skidding turn the tail is yawed toward the inside of the turn. The inside wing has a higher angle of attack. If that wing’s AOA reaches critical and stalls, the other wing is at an AOA very near that for maximum lift. The low wing drops and maximum lift on the high wing snaps the aircraft over into a spin. Intentionally flying an aircraft into this condition is the basis of a snap roll. For most of us. it is an extremely undesirable manoeuvre.
How do you maintain coordination so both wings stall at the same time? With rudder, of course. But lack of rudder coordination is only one cause of AOA asymmetry between one wing and the other. There’s another control that can severely aggravate stall characteristics and incite a spin—a hazard that, in my opinion, is not frequently taught.
Ailerons and AOA
Let’s go back to the original definition of angle of attack: the angular difference between the chord line of the wing and the relative wind. ‘Chord line’ is a line drawn from the leading edge to the trailing edge. The curvature of the wing along this line is called the wing’s camber, and that’s important to this part of the discussion.
When you deflect an aileron, you’re moving the trailing edge of that portion of the wing up and down. This changes the camber of that part of the wing, hence the angle of its chord line. Since the relative wind hasn’t changed (at least yet), the angle of attack of that portion of the wing changes. When an aileron is deflected upward it has less camber and a lower AOA. Deflected downward, it has more camber and a higher AOA. Since ailerons act in opposition—that is, when one goes up the other goes down—moving ailerons creates an asymmetry in AOA between the span of each wing that includes the ailerons.
Say you’re slowing the aircraft and/or increasing aft elevator pressure. The wings’ AOA increases. Now you add some aileron deflection—introducing asymmetry between the two wings’ angles of attack. The wing with the downward-deflected aileron may reach critical AOA and stall while the wing with the upward-deflected aileron is still generating maximum lift. When the stall breaks, the aircraft can spin toward the ‘down’ aileron. This can happen even if the rudder ball is centered—the asymmetry of AOA, and the spin, is caused by ailerons, not by rudder.
Here’s the real danger: you’re wings level and stall the wing, intentionally or not. If a wing drops, from lack of rudder coordination or propeller turning forces, the correct response is to push the elevator control to unload the wing while leveling the wings with opposite rudder. However, you naturally interpret a wing drop as a turn and the instinctive response is to turn back toward level flight—using ailerons. For example, the left wing is the one that drops. If you respond by turning the yoke or moving the stick to the right, the right aileron goes up and the left aileron goes down. The left wing’s AOA increases, sustaining the stall, while the right wing’s AOA decreases, potentially lowering it enough to return to maximum lift generation. The aircraft snap rolls toward the downward wing.
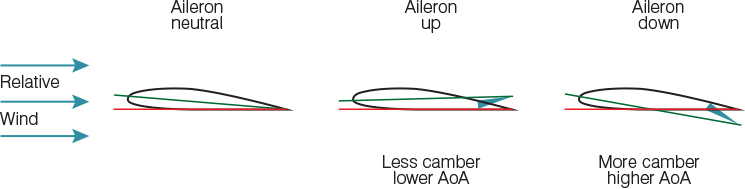
This is why it’s so important to condition yourself to keep the ailerons perfectly neutral during stall recovery. It’s not natural to do that and your every instinct is to use ailerons to raise a dropped wing. It takes practice to do this. But pushing the elevator to unload the wing, using rudder to hold or return to wings-level flight and rigidly holding ailerons neutral, must become your new instinctive response to a stall. You may get away with using ailerons in stalls sometimes, and the effect differs in one type of aircraft to the next, but the danger is always there if you use ailerons while recovering from a stall.
It’s vital not only to maintain rudder coordination at high angles of attack and during stall recovery, but also to maintain neutral ailerons in or near stalls even in coordinated flight.
Clarification
The feature ‘Rudder, ailerons, stalls and spins’ in the Autumn edition of Flight Safety Australia attracted comment from readers.
Some readers highlighted the dangers of seeking to level wings in a stalled condition by either rudder or aileron. It is critical for recovery that the first action is to push the control column forward to unstall the wings. Rudder should be used to prevent the nose of the aeroplane yawing (or further yawing) into the direction of the stalled wing and assist in the coordinated return to level flight as noted later in this article.
The following sentences attracted particular comment:
- In a slip the tail of the aircraft is displaced—or yawed—toward the outside of a turn, or to one side of a straight flight path if balanced by control input.
- By contrast, in a skidding turn the tail is yawed toward the inside of the turn.
The words ‘outside’ and ‘inside’ should be transposed in the sentences.
CASA has published a new Advisory Circular for spin avoidance and stall recovery training. The recommended procedure to recover from a stall with a wing drop is:
- apply forward movement of the control column to unstall the wing
- apply rudder to prevent the nose of the aeroplane yawing into the direction of the dropped wing
- ailerons should be held neutral until control is regained, when the wings should be levelled using coordinated inputs
- apply power and adopt an attitude to minimise further height loss. With experience, power may be introduced earlier in the recovery sequence.
- *Read the new Advisory Circular AC 61-16 Spin avoidance and stall recovery training at: casa.gov.au/ac
If the RHS wing drops in a stall, the aileron control must remain neutral and left (or opposite) rudder control should be applied to correct same!
Thanks for your comments on this topic. Some readers have highlighted the dangers of seeking to level wings in a stalled condition by either rudder or aileron. We have added an Editor’s note to clarify the recommended procedure. Also, today CASA published a new Advisory Circular for Spin avoidance and stall recovery training to provide further guidance
Read the new Advisory Circular AC 61-16 here: https://www.casa.gov.au/rules-and-regulations/current-rules/advisory-circulars
I think the slip and skid are described the wrong way around. In a slip the NOSE of the aircraft is displaced—or yawed—toward the outside of a turn (not the tail as above) and in a skidding turn the NOSE is yawed toward the inside of the turn (not the tail as above). Skids (too much rudder in to the turn) are far more dangerous than slips (not enough rudder in to the turn) so it is important to get the descriptions right. Also see https://aviation.stackexchange.com/questions/29454/what-is-a-skidding-turn-vs-slipping-turn and https://www.boldmethod.com/learn-to-fly/aerodynamics/slip-skid-stall/.
Thanks for your comments on this topic. Some readers have highlighted the dangers of seeking to level wings in a stalled condition by either rudder or aileron. We have added an Editor’s note to clarify the recommended procedure. Also, today CASA published a new Advisory Circular for Spin avoidance and stall recovery training to provide further guidance.
Read the new Advisory Circular AC 61-16 here: https://www.casa.gov.au/rules-and-regulations/current-rules/advisory-circulars
You are absolutly right with your comment.
An other thing I noticed is that the writer claims, that in a skid the inside wing has a higher AOA, and vice versa for a slip, but doesn`t explain how that comes.
While the author’s comments about avoiding the instrictive use of aileron at the stall are valid, there are some misleading comments in this article about use of rudder during stall recovery.
In particular the comment “… the correct response is to push the elevator control to unload the wing while leveling the wings with opposite rudder…” is simply incorrect. Logically, if the wings are at (or so close to) the stall that aileron cannot be used, the gross amount of rudder necessary to “level the wings”, induces a real risk of a wing drop in the opposite direction (in the direction of the applied rudder) and increases the likelihood of entry to an incipient spin.
Wing drop at the stall is invariably the result of one wing stalling before (or further than) the other. There can be a number of reasons for this, but the result is the same – roll towards the stalled (or more stalled) wing and this can be quite rapid. The correct recovery technique is to use forward elevator to unload (unstall) the wings, while preventing yaw (or further yaw) with rudder. When the wing is unstalled, aileron may be used normally to roll the wings level. Depending upon aircraft type, appropriate use of power / thrust can be used to assist the recovery.
Well stated.
Autorotation used to be described (in older aerodynamics textbooks) as a result of yaw at the point of stall.
Modern training aircraft incorporate washout in their wing design, ensuring the stall propagates from the root, and allows judicious aileron use up to the point of stall. While using aileron up to the point of stall may result in a greater adverse AoA imbalance, and exacerbate the roll instead of fix it, yaw is only a secondary effect of roll and the yaw forces required to result in autorotation are not likely to be significant. Use of rudder, to pick up a wing, is more than likely to risk autorotation as the primary effect of rudder is yaw. The complexity of the aerodynamics is that the rudder is applied opposite to the wingdrop and therefore partially serves to balance the lift/drag imbalance between up-going and downgoing wings (Better explained via lift/drag diagrams for each wing).
In some aircraft, use of rudder to pick up a wing can lead to spectacular and unintended outcomes… I have seen the world from almost inverted in aircraft varying from cessna’s to C130’s to Learjets, thanks to the flying pilot aggressive rudder use at the point of stall while attempting to maintain wings level…
I teach the basics that appear in the old aerody texts and flight instructor manuals – relax the elevator to unstall the wings, rudder to prevent yaw or further yaw, then level the wings and of course apply power and climb away. These can be almost coincidental actions, but need to be in the right order. Rudder or aileron use before the wings unstall just make things worse!
An before someone says something about minimum heightloss – the FAA have removed this from testing as it was found to be counterproductive.
Those of lucky enough to have benefited from military or advanced aerobatic training will know that you can safely manouvre an aircraft all the way up to the edge of the envelope as long as you are aware of where the envelope ends. Recovery from a g-stall can be as simple as relax your grip and allow the stick/control column forward a centimetre or two, and perhaps and gandful of thrust to increase airspeed.
Great topic of discussion!
Thanks for your comments on this topic. Some readers have highlighted the dangers of seeking to level wings in a stalled condition by either rudder or aileron. We have added an Editor’s note to clarify the recommended procedure. Also, today CASA published a new Advisory Circular for Spin avoidance and stall recovery training to provide further guidance.
Read the new Advisory Circular AC 61-16 here: https://www.casa.gov.au/rules-and-regulations/current-rules/advisory-circulars
The article is incorrect in stating that the aircraft is yawed towards the outside of the turn during a slip – in fact a slip is caused by under-use of rudder compared to balanced flight, so while the rudder is displaced on the outside of the turn relative to the balanced position, the nose of the aircraft is pointed outside, and the relative airflow comes from the inside of the turn.
Pat is quite correct is saying that at the incipient stage of the spin, before it develops into auto-rotation, the “correct” response is to move the elevator control (stick or yoke) forward and to apply sufficient anti-turn rudder to prevent further wing drop.
Once auto-rotation begins, the primary reason to use FULL opposite rudder is to help pitch the nose down to reduce the angle of attack and break the stall, as the force in rotational motion precesses by 90 degrees and it is this precession of forces that will make the wing “work” again to stop the spin.
A little-known further detail is that, for any given load (including flight loads) and CG position, there is a certain stick/yoke position at which the wing stalls, and (until auto-rotation begins) the aircraft will not stall until this position is reached or exceeded (in the aft direction).
Thanks for your comments on this topic. Some readers have highlighted the dangers of seeking to level wings in a stalled condition by either rudder or aileron. We have added an Editor’s note to clarify the recommended procedure. Also, today CASA published a new Advisory Circular for Spin avoidance and stall recovery training to provide further guidance.
Read the new Advisory Circular AC 61-16 here: https://www.casa.gov.au/rules-and-regulations/current-rules/advisory-circulars
I have just spent half an hour reading and re-reading the section on Assymetry and trying to rationalise and visualise the statements about direction of yaw in slips and skids and then consulting my BAK1 textbook from 1987. I agree with Jos and Mike that the the article has it wrong. If the article was right then a side-slip should be called a side-skid!
As a low-time private pilot in a remote area who has only resumed flying in recent years after a long hiatus, I found this confusing and potentially dangerous. I try to read as much as possible about various aspects of fixed-wing flying and I don’t have ready contact with other pilots or an instructor to discuss the finer points of aerodynamics.
Maybe I’ve got the wrong end of the stick here and if so I’ll take steps to rectify my incorrect knowledge. However if the article has got this basic detail wrong it needs to be clarified and corrected pronto.
Hi Rohan,
Thanks for the feedback. We have referred your comments to our technical team for clarification and will post a reply as soon as we can.
Clarification
The feature ‘Rudder, ailerons, stalls and spins’ in the Autumn edition of Flight Safety Australia attracted comment from readers.
Some readers highlighted the dangers of seeking to level wings in a stalled condition by either rudder or aileron. It is critical for recovery that the first action is to push the control column forward to unstall the wings. Rudder should be used to prevent the nose of the aeroplane yawing (or further yawing) into the direction of the stalled wing and assist in the coordinated return to level flight as noted later in this article.
The following sentences attracted particular comment:
• In a slip the tail of the aircraft is displaced—or yawed—toward the outside of a turn, or to one side of a straight flight path if balanced by control input.
• By contrast, in a skidding turn the tail is yawed toward the inside of the turn.
The words ‘outside’ and ‘inside’ should be transposed in the sentences.
CASA has published a new Advisory Circular for spin avoidance and stall recovery training. The recommended procedure to recover from a stall with a wing drop is:
• apply forward movement of the control column to unstall the wing
• apply rudder to prevent the nose of the aeroplane yawing into the direction of the dropped wing
• ailerons should be held neutral until control is regained, when the wings should be levelled using coordinated inputs
• apply power and adopt an attitude to minimise further height loss. With experience, power may be introduced earlier in the recovery sequence.
*Read the new Advisory Circular AC 61-16 Spin avoidance and stall recovery training at: casa.gov.au/ac
From Clarification: “The recommended procedure to recover from a stall with a wing drop is: -apply forward movement of the control column to unstall the wing…” assumes an up-right stall. An inverted stall would require the opposite. Spacial disorientation happens!
Also, all would assume in the clarification that the last bullet point should have not been bulletted, I can’t see a pilot reading the AC 61-16 whilst recovering from the stall :-) The printed version has it correctly.
An inverted stall would require the opposite. Spacial disorientation happens!
This should be stressed for everybody intending to learn aerobatics! The difference between a rightside up spin and an inverted one is marginal. The pilot feels weightless and the horizon is a blurr in both situations.
thank you for the information
Assymetry
>In a slip the tail of the aircraft is displaced—or yawed—toward the outside of a turn, or to one side of a straight flight path if balanced by control input. The outside wing is traveling faster through the air than the inside wing but is also forced into a higher angle of attack.< ???
It is suggested that the airplane begins a turn or is established in one. If opposite rudder is applied, the tail displacement will be towards the inside of the turn, reducing the rate of turn, as a result of cross controlling, i.e left aileron, right rudder renders an airplane to perform a slip.
It continues… in a skidding turn the tail is yawed toward the inside of the turn. The inside wing has a higher angle of attack.
Wrong again, the typical stall on base to final in a skidding turn is the result of too much rudder in the direction of the turn, with the tail sweeping toward the outside of the turn, making it sharper.
And now I`ll explaine how assymetry causes to always stall the wing that is on the opposite side of the deflected rudder:
Stepping into the rudder accellerates the outside wing, and decelerates the one on the inside. That instantly causes the outside wing to generate more lift than the other one.
The rise of he outside wing is, what effectively reduces its AoA, and vice versa increases it on the descending inside wing.
What we learn from this: If operating near maximum AoA, the rudder deflection and or P- factor will make the wing drop and therefore stall, on that side where the rudder is pointing to.
The use of ailerons affect the stall of the descending wing varies from airplane to airplane.